ASTR 1230 (O'Connell)
STELLAR POPULATIONS AND THE
HISTORY OF THE UNIVERSE
Stars are the building blocks of galaxies. Research on
stellar populations
is the study of the different generations of stars which make up a galaxy.
This is the principal way in which we determine the life history of galaxies.
Astronomers use the term "stellar population" to refer to a
single generation
of stars characterized by a common
age and
chemical composition.
A galaxy can be composed of a large number of individual populations, or
it can be dominated by a single generation.
We can analyze stellar populations in two main ways:
- By studying the properties of the individual stars in a
galaxy
...but this is limited only to nearby galaxies where we
can detect individual stars.
- By studying the integrated light (the combined
light output) of the stars in a galaxy
...this is less definitive, but we can apply this approach to
any galaxy in the observable universe.
This lecture describes how
astronomers are able to use integrated light to analyze galaxy histories.
A. MOTIVATION: GALAXIES IN THE DISTANT UNIVERSE
Large telescopes have provided images of thousands of galaxies at
distances over 5 billion light years. The
Hubble Ultra Deep Field is the best example of
a deep galaxy survey -- made by combining many day's worth of exposure
time with the Hubble Space Telescope. The extract from the HUDF below
shows the strange kinds of galaxies that inhabit the distant universe.
Click on the image to see the whole HUDF.
A major goal of studying very distant galaxies like these is to determine the
star formation history of the universe going back as far in
time as is possible. These galaxies are much too far away to detect
individual stars. But we can discover some of the characteristics of
the stars which make them up by analyzing their integrated light.
B. THE ELECTROMAGNETIC SPECTRA OF STARS
- Stars radiate mainly in a small region of the EM spectrum:
the ultraviolet-optical-infrared region.
- Hotter stars are bluer; they emit more of their light at
short EM wavelengths.
- Cooler stars are redder; they emit more of their light
at long EM wavelengths.
C. THE EVOLUTION OF STARS
Stars are formed
continuously in some galaxies, and mainly
in
bursts in others. In our galaxy the
star formation
rate (the total mass of gas converted into stars per year)
is about 1 solar mass per year. On the other hand, some
starbursts can reach over 1000 solar masses per year.
The combined light output of a stellar generation depends on the
temperature distribution of its stars and how that changes with
age.
The
Hertzsprung-Russell (HR) diagram is the basic tool for
analyzing the temperature distributions of evolving stellar systems.
- In the HR diagram, we plot stellar temperature (increasing
to the left) against instrinsic stellar brightness or
luminosity (increasing upward).
- Most stars fall on the main sequence (MS), which runs
diagonally across the HRD. The Sun is an MS star (yellow in the
diagram). More massive stars on the MS are brighter and hotter (hence
bluer).
- The main sequence phase of evolution is long-lived. MS stars
maintain themselves by burning hydrogen in their cores. For a star
like the Sun, that can continue for about 10 billion years because
there are huge reserves of hydrogen in all MS stars. But eventually,
those run out. When they run out of H fuel, stars evolve off the
MS (to the right, or toward lower temperatures, in the
diagram). The Sun will move toward the region marked "red giants"
in the diagram.
- More massive stars evolve faster. Even though they contain
more hydrogen. they burn it much faster than lower mass stars.
- The stars born in a given generation will have a large range in
mass. The HR diagram of that generation will change quickly as its
more massive stars evolve. Its main sequence will sequentially "burn
down" as the generation ages and the most massive stars peel off to
cooler temperatures. The mass of the stars near the
MS "turnoff" become systematically smaller with age.
- The
following animation shows how the distribution of stars in the HR
diagram changes with time. Labels by the dots indicate the masses of
individual stars (in solar units). The three panels at the right show
the structure at a given time of three stars of different mass: 2.6,
1.0, and 0.7 times the solar mass. The age is listed in billions of
years. You can see how the mass of the stars at the "turnoff" point
decreases in lockstep with the age of the generation.
D. THE INTEGRATED SED/COLOR OF STELLAR POPULATIONS
From the HR diagram for a generation of stars at different ages, we
can predict what its combined
spectral energy distribution
(SED) will be. The SED is simply the distribution of light energy
over wavelength, or color in the EM spectrum.
To predict the integrated SED of a generation at any time, we simply
add up the light of all the stars in the HR diagram, keeping
track, of course, of the fact that stars at the top of the diagram are
much brighter than those at the bottom.
A predicted SED for ages between 10 million years and 15 billion years
is shown in the animation below. The HRD of the single generation
population is shown in the lower left panel, while its integrated SED
is shown for the wavelength range 3000 to 7500 Å in the top
panel. The colored regions in the top panel show the responses for
three standard filters used by astronomers to determine the colors of
stars. The "V" filter corresponds roughly to the response of the
human eye. The lower right panel shows how the colors change
with time (blue colors in lower left, red in upper right).
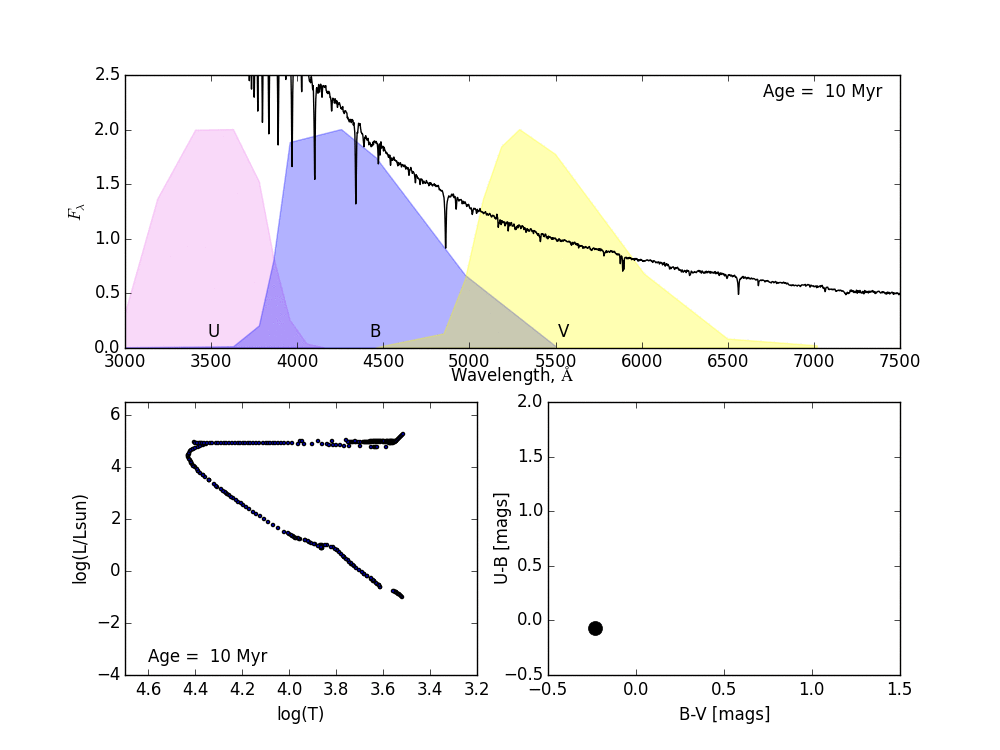
The dramatic change in the shape of the integrated SED with age is
obvious in the animation.
- A "young" (less than 100 million years age) population contains
massive MS stars. These are hot and generate much blue light.
The short wavelength SED even goes off the scale of the plot. Even
though they may contain many cool stars, young populations are blue in
integrated light
- As age increases, the SED flattens. An "old" (more than 3
billion years age) population contains no massive, hot stars, only
cool low-mass stars on the main sequence and red giants. It is
therefore yellow-red in color.
- A "very young" generation (less than 10 million years age)
produces much ionizing radiation in the extreme
ultraviolet region of the SED (not shown). This creates regions in
the surrounding gas whose spectra contain strong emission
lines of hydrogen and other ionized gases. We call these "H II"
(meaning "ionized hydrogen") regions. The dominant emission line of
hydrogen (H-alpha) has a deep red color. The combination of this
with the generally blue light of young stars produces a
characteristic pinkish tint in color images of HII regions.
.
The image of the "Whirlpool" galaxy (Messier 51) at the right
shows the pink HII regions strung out along the spiral arms.
- The sharp dips evident in the integrated SEDs are produced by
absorption by
individual
chemical species -- e.g. atomic hydrogen, calcium, sodium and iron
or molecules like CN and TiO. The abundances of these species can be
determined from the integrated SED.
The plot below contrasts two snapshots of the SED history: one of a
very young population and another of an old one, but now plotted
over a larger range of wavelength, extended into the ultraviolet band
down to 1000 Å. The
discriminatory power of the
ultraviolet for study of the SED, especially of young populations,
is exceptional, but because the Earth's atmosphere is opaque below
about 3200 Å, this can only be exploited by telescopes in
space.
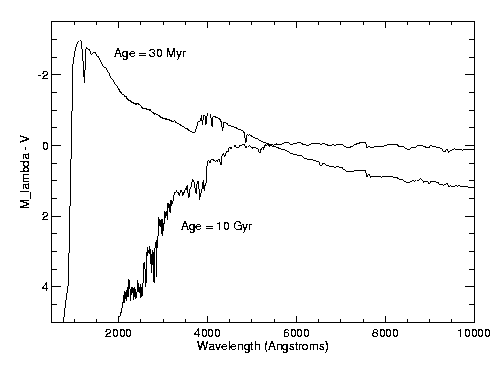
Thus, we can use
the integrated color of a stellar population to
estimate its age, regardless of how distant its host galaxy might
be. With suitable spectral resolution, we can also determine
elemental abundances for the population.
We can also estimate the
current star formation rate (SFR) of a
galaxy by using the amount of blue light it generates or the strength
of its ionized hydrogen emission lines. We do that by estimating the
total mass in young stars and dividing by their age; the result is
expressed in units of solar masses per year of new stars
being formed.
E. THE STAR FORMATION HISTORY OF THE UNIVERSE
The SED analysis techniques we've described were developed in studies
of nearby galaxies. After the
amazing images of
the distant universe were obtained by the Hubble Space Telescope,
those techniques could be applied to the galaxies detected there in
wholesale fashion.
Before that could be done, it was necessary to correct the SEDs
for the effects of the
redshift caused by the expansion of
the universe. The more distant a galaxy is, the faster it is
moving away from us. Because of the
Doppler Effect on the spectrum of receding objects, the SED
of a distant galaxy is shifted to longer (redder) wavelengths. The
amount of the shift, and hence the distance of the galaxy, can be
determined by the observed SED, and its colors can then be corrected
for the shift.
Since 1996 many studies have been made of distant galaxies, using a
combination of data from the HST, other space telescopes, and
ground-based telescopes. We now have an solid understanding of the
gross star formation history of the universe. That's displayed in the
diagram below:
This shows the estimated average rate of star formation per unit
volume on the vertical axis as a function of redshift on the
horizontal axis. The scatter of the plotted points gives a measure of
the uncertainty in the SFR determinations. The vertical scale is
logarithmic, so it is greatly compressed. The upper
horizontal scale is labeled
in
lookback time -- that
is, the time it took light to reach us from each redshift epoch. Zero
on the upper scale corresponds to the present time.
We see that the peak in the star formation density in the universe
occurred at a redshift near 2 or at an
epoch 10 billion years ago.
Star formation has dropped smoothly, by a factor of 10, since that
time. The earlier history is still sketchy because of the difficulty
of detecting galaxies at redshifts above 4, but it's clear the SFR
density was falling off above that point.
According to our best estimate, the universe is 13.7 billion years
old, so the peak in the SFR density (sometimes called
"cosmic
noon") occurred some
3.7 billion years after the Big Bang.
Much of the stellar mass that makes up the present-day universe was
therefore formed relatively early but over an extended period rather
than all at once in an intense, concentrated burst. Most galaxies
formed their initial populations at that early time, but almost all,
including ours, have continued to form stars, at a slower rate, since
then.
It's amazing to realize that we have gone from not knowing that
galaxies existed to being able to detect the first ones being
formed --- probing distances of over 12 billion light years --- in
only 80 years.
Web Links:
Last modified
December 2020 by rwo
Text copyright © 2003-2020 Robert W. O'Connell. All
rights reserved. HR diagram animation copyright © by James
Schombert (U. Oregon). Integrated SED animation copyright © by
Chris Mihos (Case Western University). Cosmic SFR history from Piero
Madau and Mark Dickinson (2014, ARAA, 52, 415). These notes are
intended for the private, noncommercial use of students enrolled in
Astronomy courses at the University of
Virginia.