ASTR 1210 (O'Connell) Study Guide
14. TELESCOPES
Summit of Mauna Kea, Hawaii
The telescope is the
single most important invention for astronomy.
It is a beautiful example of the interplay between technology
(fabrication of quality glass, optics design, polishing techniques,
large mechanical structures, computers) and basic science.
This lecture describes the main features of
optical-band
telescopes---i.e. those which operate in or near the part of the EM
spectrum to which our eyes are sensitive. This is the only kind of
telescope which was in widespread use before 1950.
Since that time, astronomers have developed other types of
"telescopes" to exploit a large part of
the
whole electromagnetic
spectrum. Cosmic sources produce radiation across the entire
range of this spectrum. Some telescopes for other spectral bands
(e.g. the ultraviolet and near-infrared) are quite similar to optical
telescopes. Others (e.g. for the radio and gamma-ray bands) use
very different technologies.
A. Introduction
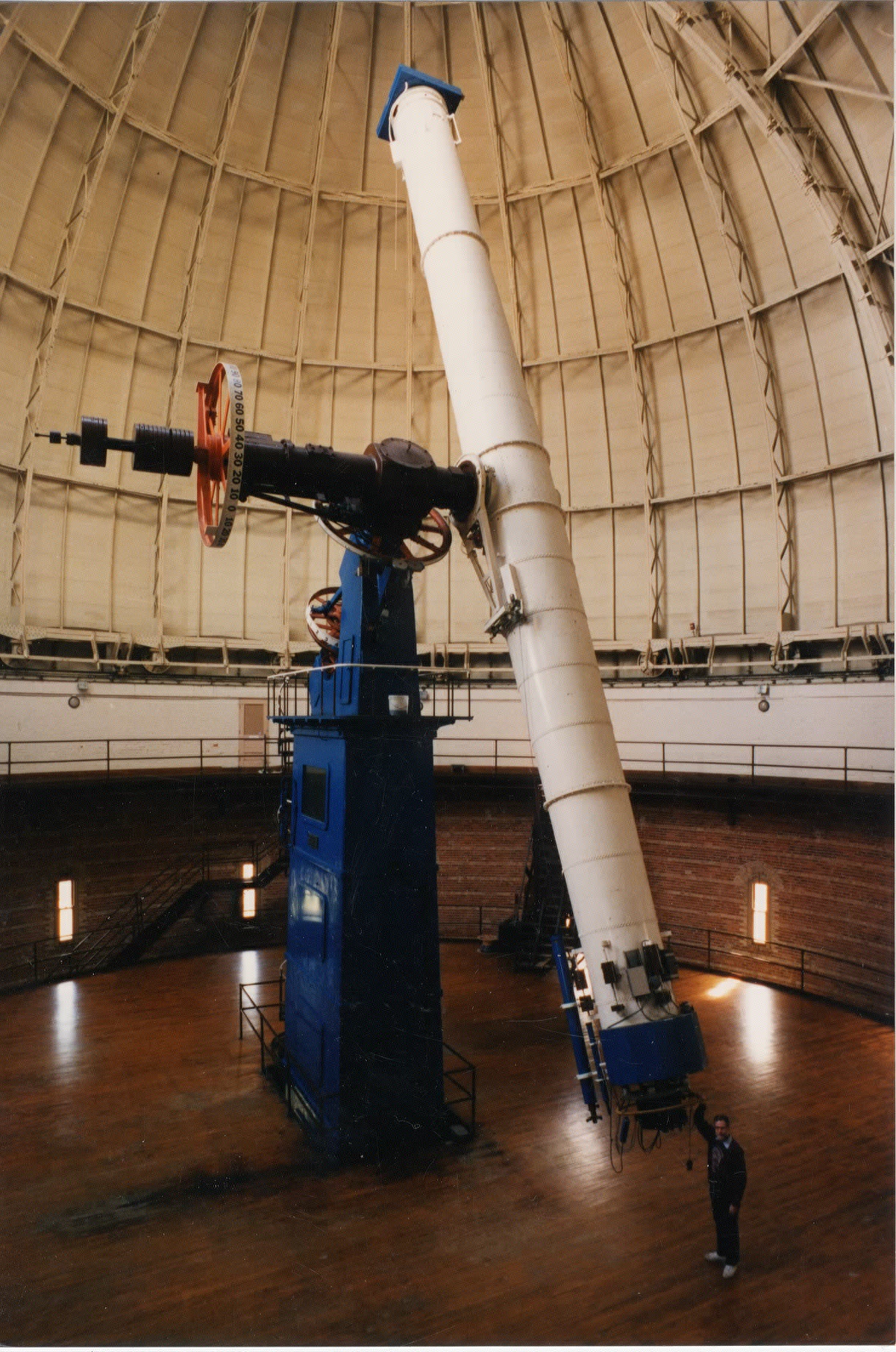
The telescope was
invented in 1608 by Lipperhey in Holland.
Although lenses had been used in eyeglasses for several centuries,
realization of higher precision optical devices suitable for viewing
distant objects depended on improvements in lens-grinding
technology. Details of the early work of Lipperhey, Galileo, and
others are
given here.
Note: the microscope was invented around 1620, also in Holland,
and was responsible for opening up a second kind of "invisible world."
Modern medicine would not exist without the microscope.
The first astronomical use of a telescope was by
Galileo, in Italy in 1609.
The telescope instantly and utterly transformed astronomy
(see
Study Guide 7).
Galileo's lenses were only about one inch in diameter and of relatively
poor quality. But telescope technology had advanced over the
subsequent 300 years to the point where the largest operating telescope
in the year 1900 was the Yerkes Observatory 40-inch telescope shown
at the right.
Purposes
- Collect more light: in order to detect fainter objects. This is the
most important function of telescopes.
- Light gathering power depends on the telescope
diameter2
- Thus, a 10-in diameter telescope collects (10/5)2 =
22 = 4 times as much light as a 5-in telescope.
- An 8-in telescope (widely used by amateur astronomers) collects
1600x more light than the human eye. Because there are many more faint
stars than bright ones, an 8-in scope can detect over 2000x as many
stars (10 million compared to 5000) as the unaided eye.
- Resolve sources better: provide sharper
images, permit seeing more detail. Resolution depends on both the
diameter of the telescope and its optical quality
- Magnify sources: make the images of distance objects
larger for easier study
B. Designs
Basic principle
- An objective or "primary" optical
element forms an image (i.e. an accurate
representation of the original scene) at a
usable focus, where it can be studied by eye, recorded
by film or other detectors (as in a camera), or fed into yet other
instruments
The objective element can be either a lens or a mirror.
Types of telescopes
There are therefore
two basic types of telescopes:
- Refracting telescopes: the objective is
a lens (shaped, transparent glass)
which refracts (or bends) incoming light rays to a
common focus. The image at the left below shows how a flat glass
surface bends light rays. In this case, two flat surfaces at a angle
have been combined to make a prism. The shorter the
wavelength of the light, the stronger the bending.
The image at the right below shows how a glass surface can be
continuously curved to bring all the light rays passing through
it from a given point on a distant object to a common focal
point.
The largest refractor ever built was the
Yerkes Observatory 40-in telescope (1896).
|
|
Refraction of Light By a Prism (click for a descriptive animation)
|
Shaped Convex Lens
|
Because refraction is stronger for shorter wavelengths, all lenses produce
chromatic aberration, in which light of different wavelengths comes
to a focus at different positions. Compound lens designs can reduce but
never eliminate the flaws in images that result.
- Reflecting telescopes: the objective is a
shaped mirror coated with a metallic film,
which reflects light rays off its front surface to a common
focus. See the picture below. Invented by Gregory (1663); improved by
Newton (1669).
Many early reflecting telescopes employed
metal mirrors, but almost all modern designs use glass mirrors.
At first sight, a reflecting design is counterintuitive because the
focus is in a position where placing equipment would interfere with
the incoming light beam. This is true, but the effects, both in terms
of blocking the light and diminishing the image quality, are small if
the mirror is large enough. Most reflecting telescopes use a
"secondary" mirror to redirect the light beam outside the main
body of the telescope. There are many different
designs for reflecting systems, each
with its own advantages and disadvantages.
Reflecting designs have a number of advantages over refractors: There
is no chromatic aberration. The mirror is easy to support from
behind, unlike a lens which must be supported from its edges and tends
to sag. Mirrors are figured on only one side, whereas lenses must be
figured on both. It is harder to support heavy lenses mechanically at
the top end of a telescope tube than a mirror at the bottom end.
Multiple mirrors can be used to fold the light beam and reduce the
length of a telescope tube.
All large telescopes are therefore reflectors. The largest
fully steerable reflector is the Gran
Telescopio Canarias (409-in in diameter: built 2009).
A telescope 1550-in (39.3-m) in diameter is
now under construction at the
European Southern Observatory.
Reflection of Light by a Figured Mirror
-
Applet. Here is a Java applet illustrating the
differences between refraction, reflection, and diffraction.
Focal plane
- For distant objects (including all astronomical objects), the
incoming rays are parallel to one another. Such rays are focussed in a
plane which is one focal length from the objective. This is
called the "focal plane." Click on
the button below for a Java applet illustrating image formation for objects
at different distances.
- In the focal plane, the light rays from a distant object form a
one-to-one representation of the distant scene which is called an
image.
- Ordinarily, a camera or other instrument in placed at the focal
plane. For "visual" use of a telescope, an eyepiece
(a smaller lens or combination of lenses) can be used to magnify the
focal plane image so it can be viewed by the eye. See the
illustration above. The magnification of the image seen
through the eyepiece is equal to the ratio of the focal length
of the objective to the focal length of the eyepiece.
C. Image Quality
The crispness of images made by a telescope depends on several factors: fabrication
of the optics, the size of the telescope compared to the wavelength of light,
and the Earth's atmosphere.
The
"resolution" of a telescope image is quantitatively defined to be
the
smallest measurable detail in an image (in seconds of arc).
Optical Figuring
- Light is a wave. In order to produce a good image,
telescope optics must be figured to a minimum tolerance of about
1/4 of the wavelength (distance between crests) of the light they
are intended to focus. For optical telescopes, this is about
10-5 cm.
- The intended shape of an optical surface, e.g. the curve in the convex
lenses shown above, must be reproduced to high precision in order to
obtain good image quality.
- Scale comparison: if a 320-in (8-m) diameter telescope mirror were
scaled up to the size of the continental United States, i.e. about
3000 miles diameter, then the maximum ripple allowed in its polishing
would be only about 2 inches!
Diffraction of light waves
Diffraction
- A fundamental limit on resolution is set by the physics of light.
Since it is a wave phenomenon,
light spreads out or diffracts when it passes through
an aperture (like ocean waves around a breakwater). This smears
out images.
Applet. Here
is a nice interactive Java applet illustrating diffraction.
- Diffraction is worse the longer the wavelength of light and the
smaller the telescope aperture. Click
here
for an illustration of how telescope size affects resolution.
- A 10-in diameter telescope with perfect optics can resolve 1
arc-sec at optical wavelengths. A 100-in diameter telescope could
resolve 0.1 arc-sec.
- Note that almost all stars are so distant that they are smaller in
angular size than 0.1 arc-sec and therefore appear as point
sources in such a telescope. Only a handful of stars can be
resolved by even the largest telescopes.
"Seeing" Produced by Earth's
Atmosphere
"Seeing"
- The Earth's atmosphere also refracts light; and
because it is constantly moving, there is always a blurring and
jittering of images in a telescope. Astronomers call this "seeing."
Seeing actually dominates diffraction in most cases and usually limits
telescope resolution in practice to 0.5-2 arc-seconds.
- Above is an enlarged image of the bright star Betelgeuse seen
though a large telescope. It is a large blob, broken up into smaller
near point-like units. Click on the image for a
video of the seeing effects. In
the absence of the atmosphere, the image would be steady with all of
the light concentrated into one of those point-like structures.
- To overcome seeing effects, special equipment such as
adaptive optics, which can sense and partially correct
for atmospheric blurring, can be used. Or, telescopes can be placed
in space (where there's no atmosphere), though this is much
more costly.
Sky Background
The natural nighttime sky in directions away from bright sources is
not totally dark.
There are several kinds of pervasive, diffuse
"background" light: scattered sunlight or moonlight in the Earth's
atmosphere, airglow
emission from the atmosphere's upper layers, scattered light from dust
in the interplanetary and interstellar
medium, and faint stars and galaxies.
Sky background light does not blur images, but it certainly interferes
with detection and study of faint sources (and there are many more
faint sources than bright ones).
Compounding the natural background is
manmade light
pollution, a problem with which astronomers have wrestled for
centuries. Initially, telescopes were built adjacent to universities,
usually in or near cities. However, the
growth of
artificial light pollution drove telescope construction to ever more
remote sites, first in the southwestern US (e.g. California, Texas,
Arizona), and finally to high mountains like
Mauna Kea in Hawaii or the northern deserts of Chile.
Ultimately, astronomers have placed telescopes in space to escape the
effects of atmospheric backgrounds altogether (see next
section).
Mirror blank for one of the two mirrors of the Large
Binocular Telescope.
Click for enlargement.
D. Current Telescope Milestones
The Hubble Space
Telescope: 94-in diameter reflector in orbit around the Earth
(launched 1990)
HST is not a large telescope by modern standards. But it has
produced the highest resolution images yet obtained at optical
wavelengths, with blur sizes of only about 0.05 arc-seconds. This is
because of its high quality optics and the fact that it
is outside the Earth's atmosphere, so it does not have to
contend with seeing or absorption or scattering by atmospheric
constituents. Its high resolution and the absence of the natural
and artificial atmospheric background also allows it to
detect very faint
sources.
Here is a composite
version of some of HST's best images.
Keck Observatory:
Two 400-in "segmented mirror" telescopes (1993, Hawaii). The
collecting area of each consists of 36 independent 36-in hexagonal
mirrors. See image at right and
this diagram.
The Very Large Telescope (VLT): Four
320-in monolithic mirror telescopes (2001, Chile)
The Large Binocular Telescope: two 330-in (8.4-m) diameter
monolithic mirrors on a common mount, providing the largest existing
collecting area. One of the mirrors is shown above. UVa is a partner
in this project.
The James Webb Space
Telescope, was launched on Christmas Day in 2021.
JWST is the follow-on to HST. It features a 255-in (6.5-m)
diameter primary mirror (a 25 square meter collecting area, 5.5 times
that of HST) composed of 18 hexagonal segments that had to be deployed
on-orbit. JWST carries four imaging and spectroscopic instruments and
is optimized for the near-infrared spectral region. It achieves the
same spatial resolution in the NIR spectral band as HST does in the
optical band. A large sunshield permits a low overall structural
operating temperature through cooling to space. JWST orbits
around the Earth-Sun L2 point, about 900,000 km from
Earth.
See this
graphical comparison of the collecting areas of the largest
existing and planned telescopes.
Large mirror technology
Here (from
the Magellan
Observatory) is a brief description of how the latest
generation of huge telescope mirrors are manufactured:
The Magellan main mirrors are f/1.25 paraboloids and a radical
departure from the nearly solid-glass mirrors of the past. Each is
21,000 pounds of borosilicate glass with a lightweight honeycomb
structure inside. It took 6 months to build the mold for each mirror,
2 days to fill it with chunks of glass, 1 week to melt the glass and
spin it into shape (in a specially designed rotating oven), and 3
months for the glass to cool. Each was then polished for 8 months
while its surface was constantly tested for accuracy. Relative to
their size, the main mirrors are about as thin as a dime.
The aluminum surface of each mirror is a mere four-millionths of an
inch (0.1 micron) thick. Each also sits in a "cell" that peforms two
important functions. First, the cell's thermal control systems prevent
warping from thermal expansion and contraction. Second, the support
systems in the cells maintain the mirrors in their proper shape, so
there is no distortion or cracking. The actual shape of the mirror
surface is controlled to within two-millionths of an inch (0.05
microns).
Other EM spectral bands
The telescopes we've discussed so far operate only in the optical
(or "visible") and adjacent spectral bands, but astronomers now
exploit most of the
full electromagnetic spectrum to observe the cosmos. The
first instruments outside the visible range were radio telescopes
(1950's). Now astronomers operate not only radio telescopes
(e.g.the National Radio Astronomy
Observatory, with headquarters in Charlottesville) but also
microwave, infrared, ultraviolet, X-ray, and gamma-ray telescopes.
All of the devices for detecting EM waves are called
"telescopes," even though some (e.g. radio antennas) look nothing like
classical optical telescopes.
Because the Earth's atmosphere screens out many parts of the the EM
spectrum (see Study Guide 10),
telescopes for the gamma-ray, X-ray, ultraviolet, and parts of the
infrared and microwave spectrum must be placed on spacecraft outside
the atmosphere.
E. Next Generation Telescopes
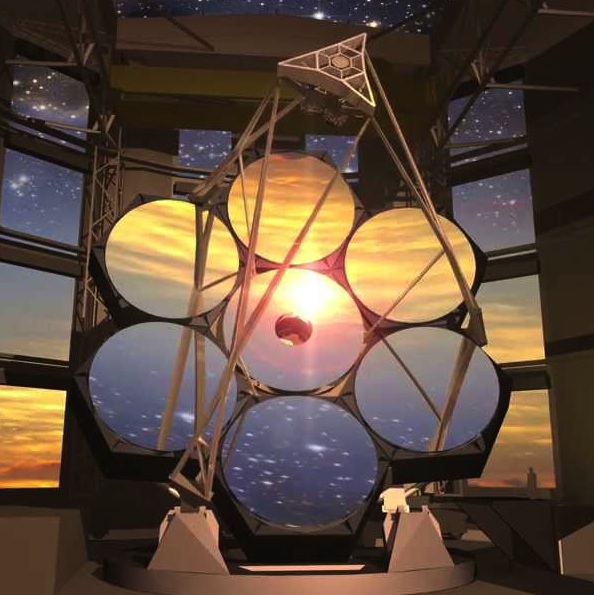
Two very large telescopes based on the segmented-mirror concept
of Keck have been designed:
the
Thirty Meter Telescope
and the
European
Extremely Large Telescope (39-meters or 1550-in). The ELT is now
under construction in Chile, but the TMT is embroiled in a dispute
over environmental and cultural impacts at its preferred Mauna Kea
site. The
Giant Magellan Telescope
(at right), also
under construction in Chile, is a multiple-mirror design with
7 8.4-meter spin-cast mirrors and an equivalent collecting area
of a single 22-meter mirror. The six off-axis segments are
challenging to figure to the correct surface shape.
Another innovative telescope with a very different design and
operations mode is the
Large
Synoptic Survey Telescope of the Vera C. Rubin Observatory. To
achieve a wide field of view (3.5
o) it employs a unique
3-mirror design in which the primary and tertiary mirrors have been
figured on a single piece of spin-cast glass 8.4-meters in diameter.
The telescope is intended to repeatedly image the entire usable sky
every three nights, searching for transient or moving targets
(including near-Earth asteroids) while building up an ultra-deep
combined image of the sky. Continuous output from its 189 imaging
CCDs (see below) will generate an unprecedented data volume (15
TB/night). Under construction in Chile, LSST should be in operation
by 2024.
F. Detectors
The
human
eye is a sophisticated, auto-focus, auto-exposure, electrical
camera system. However, for all its versatility and importance to us
in everyday life, it is a seriously limited astronomical detector: it
is small, its maximum integration time is only about 0.1 sec, and it
has low sensitivity. Astronomers have long sought more capable
detectors to use with telescopes. Descriptions of the two most
important kind of imaging detectors are given next:
Photographic Film
- Film was the main astronomical detector used between 1900 and 1980.
- It detects only 1-2% of incident photons (not much better than
the eye) but allows long integrations (hours)
- Requires chemical development of image after exposure, a
serious complication.
- Provides permanent storage of image information---a tremendous
benefit. But information on film is not in digital form, which makes
quantitative analysis difficult.
- Extends the observable EM wavelength range to regions (the
near-UV and near-IR) where the eye is not sensitive
- Large formats are possible (up to 20-in square for astronomy)
- Nonlinear, nonuniform response makes quantitative measures of incident
light energy difficult
Charge-Coupled Device Architecture
"Charge-Coupled-Devices" (CCD's)
- CCD detectors are a type of solid state electronics, using
a light-sensitive silicon wafer fabricated with embedded
microelectronic integrated circuits
by photolithography.
.
- See image above. The CCD surface is composed of millions of
independent, light-sensitive pixels.
- After exposure, pixel
contents are shifted in 2 dimensions across the surface to an output
amplifier and storage device.
- Astronomical applications were pioneered during development of
the Hubble Space Telescope (1974-85).
- CCDs work well at both very short (TV) and very long (astronomy)
exposure times.
- They are 50-100x more
sensitive than film
- They provide digital image storage for immediate computer
processing
- Determination of incident light energy is easier
- Only small
formats are available (2-in typical); but one can "mosaic"
CCDs to create a large area detector surface
- CCDs are now the standard detectors used in optical-band astronomy.
CMOS solid
state detectors, with characteristics similar to CCDs, are
employed in commercial still and video cameras and cell phones. These
are mass-produced in quantities of billions. They are significantly
less well suited to astronomical use (being harder to calibrate, for
example), but astronomical applications are now being explored.
Many other types of electronic detectors are now used in the UV, IR,
and X-Ray bands of the astronomical EM spectrum.
Sunset over the William Herschel Telescope (La Palma,
Spain; N. Szymanek)
Reading for this lecture:
Study Guide 14
Bennett textbook Chapter 6
Reading for next lecture:
Bennett textbook: pp. 203-204; Secs. 9.3, 9.5.
Study Guide 15
Web Links:
Last modified
June 2023 by rwo
Text copyright © 1998-2023 Robert W. O'Connell. All
rights reserved. These notes are intended for the private,
noncommercial use of students enrolled in Astronomy 1210 at the
University of Virginia.