ASTR 1210 (O'Connell) Study Guide
11. PLANETARY SYSTEMS: OURS AND OTHERS
Comparison of the planets, based on
NASA images.
Sizes are to scale, but separations are not.
The four large satellites of Jupiter discovered by Galileo in 1610
with his small telescope were the first new members of the Solar
System identified in recorded history. They instantly increased the
known membership of the Solar System from 8 to 12. Since that time,
astronomers have identified hundreds of thousands of Solar System bodies
(planets, satellites, and smaller rocky or icy objects) with telescopes
and spacecraft.
Most remarkably, after thousands of years of speculation about other
worlds like ours in the universe, astronomers have recently discovered planets
in orbit around other
stars---exoplanets.
This lecture describes the general properties of our planetary system and
those around other stars and how we believe these originated.
A. Inventory of the Solar System
By terrestrial standards, the density of matter in the Solar System is
extremely low, and the planets are separated by enormous gaps.
Contents of the Solar System:
- The Sun (99.8% of total mass)
- 4 "terrestrial" (Mercury, Venus, Earth, Mars) and 4 "Jovian"
(Jupiter, Saturn, Uranus, Neptune) planets (0.1% of the mass).
Jupiter's mass is greater than the masses of the other planets
combined.
- Over 180 satellites of planets
- Hundreds of thousands of smaller rocky or icy bodies, ranging in
size down to about 50 meters. The largest of these, including Pluto,
are now called "dwarf planets." (We will postpone discussion
of the controversy over the use of this terminology
until Study Guide 20).
Most of these are
members of the asteroid belt between Mars and Jupiter or the
"Kuiper Belt" in the outer solar system, beyond Neptune.
Objects smaller than dwarf planets but larger than about 50-m
diameter are called "asteroids" if they are composed
of rocky materials or "comets" if they are icy.
- Meteoroids (rocky or icy bodies smaller than
50-m), dust, gas
- Other than the Sun, no solar system object is self-luminous at
visible wavelengths, and all shine by reflected sunlight. Seen
from the Earth, the second and third-brightest celestial objects are
Earth's Moon and Venus.
For a diagram of the current location of the planets in their orbits,
click
here.
An oblique view of the planetary orbits drawn to
scale
(though the planet sizes shown are not to
scale).
B. Systematics of Planet Orbits
Systematic characteristics of the orbits of the terrestrial and Jovian
planets:
- Orbits for all lie close to the plane of the
Earth's orbit (the ecliptic plane)
In the picture above, you can see the "cozy" inner solar system
(Mercury through Mars) separated from the vast outer solar system by
the asteroid belt.
For an edge-on plot of the orbits showing the near-coincidence of the
orbital planes, click here.
The orbits of the "dwarf planets," including Pluto, can be more highly
inclined to the ecliptic plane.
- The orbits, though technically ellipses, are nearly circular
- The direction of revolution of the planets in their orbits is the
same (counterclockwise from above Earth's N. pole); the direction of spin
on their rotation axis is the same for most.
- The orbits show systematic spacing (Bode's "Law"): the
separation between orbits increases with orbit size from Mercury
through Uranus. This is apparent in the figure above.
It is important to understand that
none of the above is required by
Newton's laws.
For example: Newton's laws imply the orbit of a given planet will be in a
fixed plane, but the orbits of other planets could lie in different
planes; revolution directions do not have to be the same; orbits can
be highly elliptical rather than nearly circular; etc.
Instead, these systematics must be the product of
special
physical conditions prevailing during formation of the planets. That is,
they provide clues to the process that forms planets.
C. Segregation of Physical Properties
The four
"inner" or "terrestrial" planets (Mercury, Venus,
Earth Mars) show a striking dissimilarity from the four large
"outer" or "Jovian" planets (Jupiter, Saturn, Uranus, Neptune):
|
INNER (TERRESTRIAL) |
OUTER (JOVIAN) |
Size & Mass** |
Small |
Large |
Density |
Large |
Small |
Composition |
Si,O,Al,Mg,Fe Rocky |
H,He Gas Giants |
**See the image at the top of this page for a graphic comparison
of planet sizes.
These differences constitute another
major clue about the
processes that formed the planetary system.
Note: we are deliberately omitting Pluto here, since it is not
representative of either group.
D. Origin of the Solar System
Since the time of Galileo, there have been many models for the origin
of the solar system. They all fall into two main categories:
- "Catastrophic/tidal theory": a passing star pulls material from Sun, which cools
to form planets
This would imply planets are rare, since close encounters
between stars are extremely rare.
The process is also physically unlikely: expelled solar material would
be very hot and would diffuse quickly before it was able to
condense.
- "Nebular theory": planets form from the cloud ("nebula")
of cool debris surrounding a forming star. The theory was first
described in 1755 by philosopher Immanuel Kant.
This would imply planets are common because they are byproducts
of normal star formation.
There is now excellent supporting evidence for the nebular theory,
not least of which is item "G" below
E. The Interstellar Medium and Star Formation
We know that
stars are forming
continuously out of the "
interstellar medium"
at a rate of about 1 solar mass per year throughout our Galaxy:
- The interstellar medium (ISM) is the dilute matter between stars.
It constitutes a few % of the mass of our Galaxy
- Including the whole volume of the disk of the Galaxy, the average
density of the ISM is about 1 atom per cubic centimeter.
- Contents of the ISM:
Gas (atoms, molecules)
Composition: hydrogen 71%; helium 27%; all other elements (C,N,O,Fe,
etc) only 2%. When hot, or "ionized," this gas is visible as
"gaseous nebulae"
(producing optical spectra containing, among other features, bright
emission lines of hydrogen, as discussed
in the Supplements). Where the gas
is cold, and in atomic or molecular form, we can detect it using
radio telescopes.
"Dust"
Very small solid particles
or "dust grains", mostly
formed in the extended outer atmospheres of cool "red giant" stars
and ejected into interstellar space. These are smoke-like. They
absorb and redden light passing through them. Absorption by
concentrations of dust creates
"dark clouds" seen
against bright background sources such as the Milky Way.
Dust plays the essential role of a refrigerant for
interstellar gas. Parts of the ISM, if well shielded by dust grains
against heating by surrounding stars, can become very dense and
cold (reaching only about 10o K above absolute zero
temperature). These are the regions which are primed to turn into
nurseries for newly born stars.
A beautiful example of a likely stellar nursery is shown in
the picture below:
This is the "Eagle Nebula" imaged by the Hubble Space
Telescope. The extended, dark, sculpted "elephant trunk" running
across the image is a cold, dusty region. It is surrounded by hot gas
(greenish-blue), which is evaporating the cold material away. The
small globules on the end of the finger-like protuberances are the
densest regions of the cloud, possibly containing protostars with
masses like the Sun. Click on the image for a full view. For more
pictures and information, click here.
|
here is an MPEG video of a zoom into
the Eagle Nebula.
For Hubble Space Telescope images of another spectacular star-forming
region in the Carina
Nebula, click
here.
F. Planet Formation in the Nebular Theory
Star formation out of the interstellar medium is an amazing process.
The compression of the dilute ISM into the body of a star occurs
because of the combined effects of gas cooling, which reduces the
thermal pressure that keeps clouds inflated, and relentless
gravitational attraction. The compression in volume is an astonishing
factor of about 10
24. The mass that ultimately resides in
a star like the Sun originally occupied a volume about 10 light years
on a side, a factor of 100 million larger across.
We now have a good, general understanding of how stars and planets
form. The detailed physics and chemistry of the processes involved
are still being worked out, but progress over the next decade or so
should be rapid because of powerful new observational facilities like
the
Atacama Large Millimeter Array (ALMA, a radio
telescope, which can detect molecules and dust grains in planetary
systems) and the
James Webb Space Telescope
designed to attack these specific problems. ALMA is operated by the
National Radio Astronomy Observatory here in Charlottesville. JWST,
the successor to the Hubble Space Telescope, was launched in 2021.
Here is the sequence of steps in the formation of stars and
their planetary systems:
- Gas in an interstellar cloud cools down and slowly compresses.
Dust grains shield the interior and allow it to fall to very low
temperatures. After a certain point, a dense, cold cloud in the
ISM will start to collapse faster under its own self-gravity:
- As it collapses, the cloud spins up & flattens because of
the conservation of angular momentum (first illustrated by
Kepler's Second Law).
A rotating, flattened "protoplanetary" disk is a natural
consequence of the collapse of the interstellar cloud and is expected
to accompany star formation in all enviroments. In the case of our
solar system the disk is called the "solar nebula." In the densest
parts of the disk, dust grains grow in size and begin to accumulate
into larger solids.
Note! The scale of this picture is much smaller, by
several 1000x, than the scale of the previous picture.
- The dense concentration of material in the center of the disk is
the "protostar" ("protosun" in the illustration here).
The protostar heats up, first from energy released by
gravitational collapse, and later, once its core reaches a critical
density and pressure, by nuclear reactions. The generated heat
counterbalances the self-gravity of the protostar and prevents it from
collapsing further.
- The protostar heats the inner protoplanetary disk to
a higher temperature than the outer disk.
Here is a typical
temperature profile for the disk. Because material in the disk is so
dense, large parts of the disk can remain very cold for some time even
if the inner regions are quite hot.
- The heating determines the kinds
of solids which
can survive in a given part of the disk and generates
the segregation of planetary properties:
Only "refractory" (high melting point) solids survive in
the inner disk. These tend to be heavy, rocky materials. Only
a small fraction of the total inner disk is in this form since
heavy elements are not abundant.
"Volatile" materials are those with low melting points.
They include the ices of water, methane, and ammonia (H2O,
CH4, NH3). These will be vaporized in
the inner disk.
On the other hand, these ices can persist in solid form in the
cool outer disk. These are hydrogen-rich compounds, and because
H is abundant, there is a large amount of such icy material in
the outer disk.
The innermost radius in the disk where icy materials can remain solid
is called the "frost line".
Solids in the outer nebula are more similar in chemical
composition to the Sun than are inner nebula solids
- Larger bodies grow initially from the solids, not from
gas, through collisions and sticking together
(or "accretion").
Accretion produces solid bodies with a range of sizes in the sequence
grains ==> pebbles ==> "planetesimals" ==> "protoplanets", where
the distinction in size between the two latter classes is not firmly
defined. A protoplanet is an object over about 500 km in diameter.
For larger protoplanets (about 15x the mass of the Earth),
gravitational fields can rapidly attract gas from the nebula.
In the inner disk, small, rocky proto-planets form.
In the outer disk, beyond the frost line, large, "gas-giant"
proto-planets form.
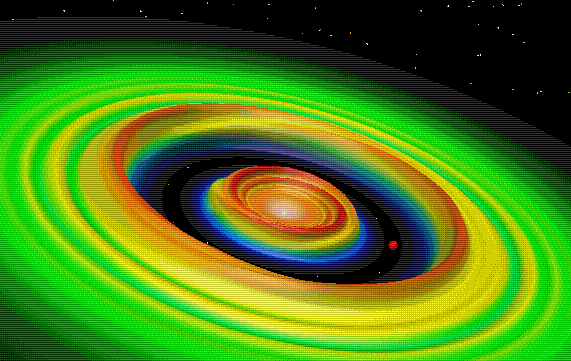
Computer simulation of protoplanetary disk
- Final assembly: the violent infall of fragments heats
the protoplanets. Collisions
between protoplanets and large fragments can have drastic effects,
producing extensive melting/resurfacing in a merged protoplanet or
even shattering the originals into smaller pieces.
- The elapsed time for proto-planet formation is now thought to
be very short by cosmic standards, ranging from a few hundred
thousand to a few million years.
- The interiors of proto-planets that are large enough are heated
and partially melted by the violent accretion and by the
decay of short-lived radioactive isotopes. The melted interiors will
differentiate, with heavy metallic materials settling
to the center and lighter, rocky materials rising to the exterior.
- Gravitational interactions between planets, or between planets and
the residual protoplanetary disk (as in the image above), can
drastically change the orbits of the new planets, moving large
planets inward, tossing small bodies outward, or pushing planets into
more strongly elliptical orbits.
- Here is a pictorial
summary of the nebular model.
Observational Support for the Nebular Model
- The nebular model successfully explains the systematics in the orbital
geometry, motions, and compositions listed in Sections B and C
above.
- We now
have direct detections of protoplanetary disks around nearby
stars by
the
Hubble Space Telescope,
infrared telescopes, and
ALMA.
A montage of disk images made in the far-infrared bands, where cool
dust glows brightly, is shown above. The physical and chemical properties
of these disks are now being studied intensively with ALMA and other
sensitive instruments.
- The expectation from the nebular model that planets should
be common and that most stars should have accompanying
planetary systems has now been confirmed in dramatic fashion by the
discoveries described in the next section.
G. Exoplanets
Speculation about
planets around other stars extends as far
back in history as the ancient Greeks. The philosophical implications
of discovering other planetary systems for the context in which we
should view the Earth and the human race have been widely discussed.
But for hundreds of years after the invention of telescopes, no
planetary companions to other stars were found despite much
effort.
Finally, extra-solar planets around other Sun-like stars were
positively
detected in October 1995. ("Extra-solar" means
planetary systems other than that around the Sun, now usually
abbreveviated simply to
"exoplanets".) We have not
only detected exoplanets, but we have established that they
are
relatively common around Sun-like stars in the Galaxy.
And, using special techniques and highly sensitive detectors, we have
begun to probe the composition and structure---even the
meteorology---of some exoplanets.
Awarding credit for the discovery of exoplanets is, as in other
famous cases in astronomy, complicated. The first report of an
exoplanet that was ultimately confirmed was made by Campbell, Walker
and Yang in 1988. But the confirmation did not come until 2002. In
1992, radio astronomers Wolszczan and Frail detected two exoplanets in
orbit around a neutron star (whose pulsed radio emission allows high
precision measures of stellar velocity). Whether these are "normal"
planets or the product of the tremendous supernova explosion that
produced the neutron star has yet to be established. The detection by
Mayor and Queloz of the first Jupiter-like exoplanet (51 Pegasi b)
around a normal, "main sequence" star like the Sun was announced in
1995.
Regrettably, the Nobel Prize, which is certainly justified in this
case, can be awarded to a maximum of three scientists -- and
in October 2019 it was won by Mayor and Queloz.
Detection Methods
The initial detections of exoplanets were technically
very
difficult (or they would have been found sooner!).
We cannot simply take a picture and
see the planet. The
images of the star and planet are blended together in an
ordinary telescope (see the discussion of telescope "resolution"
in
Study Guide 14), and the feeble
reflected light of the planet is
completely overwhelmed by the bright star.
Instead, several sophisticated methods have been been developed for
finding exoplanets
(see
this article). We discuss below
only the two most widely used
of those. The "Doppler method" is particularly important because it
is the primary means by which we can obtain estimates of an
exoplanet's
mass.
Both of these techniques are
biased in the sense they are much
more sensitive to
larger planets at small distances (say
less than 1 AU) from a star.
Doppler Velocity Method
The Doppler method for finding exoplanets is based on
detecting the tiny changes in the star's
motion induced by the gravity of an orbiting planet.
These amount to only a few 10's of meters per second.
Recall that in Newtonian gravity, a planet exerts the same force on
its parent star as the star exerts on the planet. The star therefore
accelerates in response, though the acceleration is very
small because it is in inverse proportion to its mass. The animation
at right shows how their mutual gravity causes both the star
and its planet to move around a common "center of gravity." (The
stellar wobble in the animation is greatly exaggerated compared to the
motion induced by normal planets.)
The motions can be detected by the Doppler effect on the
spectrum of the star (see
the Supplements),
although special
spectrographs, with high sensitivity and stability, are
required.
Shown at the right is the Doppler-derived velocity curve
(velocity plotted against time) for the parent star of the first
extra-solar planet identified around a Sun-like star (by Mayor and
Queloz). Click for an enlargement. The amplitude of the velocity
change is +/-50 meters/second, but the precision of the data is better
than 10 meters/sec--- a velocity that can be achieved by a fast
sprinter. So we can now detect stars moving at human
speeds!
The shape of the velocity curve allows us to determine the
planet's orbital shape. A markedly elliptical orbit
will produce an asymmetrical curve.
By applying Kepler's Third Law
to the observed orbital period and velocity amplitude, this method
provides an estimate for the mimimum mass of the planet. (It
is a minimum because we usually do not know the inclination of the
plane of the planet's orbit to the line of sight; a tilt of this plane
will reduce the observed radial velocities.)
Transit Method
After a number of planets were detected by the Doppler technique,
astronomers realized that they could also find them by searching
for planetary transits, that is, the very tiny change in light
from a star when a planet crosses its disk seen from our point of view
(i.e. a partial eclipse). In principle, astronomers could have
detected these with many of the electronic devices available since the
1950's---but they had never configured their observing techniques to
look for effects this small.
For a diagram showing the viewing configuration for a
transit, click here.
The picture at the right shows a typical "light-curve" for a planetary
transit --- i.e. the observed brightness plotted against time. Note
that the amplitude of the eclipse shown here is only 1.5% of the
star's normal brightness.
This method can catch only the small fraction of planets whose orbital
planes have the right orientation such that eclipses occur from the
Earth's point of view. However, with stable and sensitive digital
cameras, it has proven possible to detect many transiting planets even
with modest-sized telescopes. Many groups around the world, including
amateur astronomers, are now surveying the sky for planet transits.
Normally, a transiting detection is not counted as a confirmed planet
until a corresponding Doppler detection is made. However, in the case
of systems with multiple transiting planets, the various phenomena
that can lead to false positives can be confidently ignored,
and the transiting objects are regarded as validated.
Aside from identifying new planets, the transit method is invaluable
because, by determining the fraction of the parent star's surface that
is occulted, it provides an estimate of the radius of the
exoplanet. (We know the sizes of stars based on our understanding of
their internal and atmospheric physics.) It also allows precision
timing of exoplanet motions in their orbits and changes in those.
The Kepler Mission
Kepler was a small space telescope carrying a huge electronic
camera (42 CCD detectors) which continuously monitored a single large patch
of sky in the Milky way between Lyra and Cygnus searching
for planetary transits in the tens of thousands of stars
observable in the field. Planetary systems identified here are very
distant (typically over a thousand light years away), but the strength
of the mission was that it could potentially find a large number of
planets. The Kepler survey field is shown at the right.
Kepler was launched in 2009 and identified thousands of
planetary "candidates" --- but most of these must be
confirmed by the Doppler velocity method before they are accepted as
planet
detections. By 2017, Kepler had found 5011 candidate
planets, 2512 of which had been confirmed.
By design, Kepler was able to detect the very tiny changes in starlight
(0.01%) caused by eclipses by Earth-sized planets. It
identified scores of candidates with sizes less than 3 Earth radii.
In May 2013, Kepler lost a key component of its attitude-control
system and its ability for precision pointing, regretfully just as it
was exploring the Earth-like planetary regime around Sun-like stars in
both size and orbital period. However, some clever engineering
innovations to stabilize the spacecraft made it possible to continue
the mission while observing fields other than the original one. Kepler
made a number of additional exoplanet detections before it
ran out of fuel in October 2018 and the mission had to be retired.
TESS
The Transiting Exoplanet Survey Mission
(TESS),
an orbiting imaging telescope, was launched in April 2018 and will
continue the space-based search for transiting exoplanets begun by
Kepler. It will survey most of the sky but only for periods of 27
days per field. It will emphasize study of about half a million
nearby stars and especially types of stars significantly smaller than
the Sun ("red dwarf" stars), where it is easier to find planets in
the "habitable zone".
Exoplanet Count
As of 2020 we had found over 4300 confirmed planets in over 3100 planetary
systems. 700 of these are multiple planet systems. Over 3000 are
transiting planets.
For a complete list, see the
Extra Solar Planets Encyclopaedia.
Properties of Important Types of Exoplanets
Hot Jupiters: "Hot" Jupiters are gas giant planets (~ Saturn
mass or larger) in very small orbits---less than 0.4 AU,
the radius of the orbit of Mercury. The tightest orbits are
less than 0.01 AU in radius. Hot Jupiter atmospheres are heated
to high temperatures by their parent star; many are puffed-up
or losing material. Under some circumstances, the atmospheres
may evaporate altogether.
This was the first class of exoplanets to be discovered around
Sun-like stars because they produce large Doppler signals. However,
they were a great surprise because in our solar system the
large planets are all at distances greater than 5 AU, and it is
thought (see item F.6 above) that they cannot form at small
distances from their parent star. Probably, these planets do form
at large distances but, because of gravitational interactions with the
protoplanetary disk, they migrate over time nearer the
star.
Because early planet searchers thought massive planets
would always be distant from their parent stars, with long orbital
periods, most did not look for short-period oscillations of the stars
(until after the 1995 detection).
[Note: objects with masses larger than 13 x Jupiter's are considered
"brown dwarf" stars, not planets.]
Super-Earths: These are exoplanets with masses significantly
larger than Earth's but smaller than 10 Earth masses. They are
smaller than Uranus or Neptune. There are none in the Solar System.
Scores of these have been detected so far around other stars. The
exoplanet system Kepler-62 has four Super-Earths. Some Super-Earths
have masses and radii that suggest they are made of rocky materials,
like Earth, or of ice/water. A number are in or near their
star's habitable zone, where
surface temperatures allow the presence of liquid water. Water vapor
and clouds were detected in the atmosphere of one such Super-Earth
(K2-18b) in 2019. But its hydrogen-rich atmosphere makes it
unlikely to host a biosphere.
Earth-Like Planets: A number of Earth-sized candidates
have been found by the Kepler mission. In April 2014, Kepler
announced the confirmation of Kepler-186f, the first Earth-sized
planet in its star's habitable zone. K186f is 11% larger than
Earth and orbits a so-called "M dwarf" star about 500 light years away
with a period of 130 days. Because its star is fainter than the Sun,
the small (0.4 AU) orbit of K186f places it within the habitable
zone.
Another tantalizing Earth-mass exoplanet in the habitable zone
is Proxima
Centauri b, which was detected by the Doppler method in orbit
around a small, red dwarf star. In this case, however, it happens to
be the star nearest to the Sun (only 4 light years away), so
the planet instantly became a target for extensive exploration.
(Proxima Centauri is part of the triple-star system Alpha
Centauri -- the third brightest star in the sky.)
The champion system for Earth-like planets was announced in February
2017, based on transit data from the Spitzer Space Telescope and
terrestrial telescopes. It is
"TRAPPIST-1", a
very small, cool star, 36 light years away, which hosts a total
of seven Earthlike planets in or near the habitable zone. The
orbital periods range from 1.5 to 20 days. Spectroscopic searches for
evidence of water in the atmospheres of the planets are already
underway.
How Many Planets in the Galaxy?
Based on the excellent statistics from Kepler, astronomers estimate
there are 100-400 billion planets in our Galaxy, though again most of
these are not Earth-like nor in a terrestrial habitable zone. There
are probably at least as many planets in the Galaxy as there are
stars.
Think about that the next time you look at the sky on a clear
night or a deep image of a
Milky Way star field.
Exoplanet Art
The discovery of exoplanets inspired an explosion of artists'
impressions of exoplanet systems and surfaces. Some nice ones are
shown in the composite below. Bear in mind that no telescope is
powerful enough to produce images like this, so they are all entirely
speculative. Almost all contain some unphysical features (like
showing the shape of other planets in the parent system).
Reading for this lecture:
Alert! Most lectures from now on will cover more material in the text
than has been the case up to now. Try to keep up with the reading.
Study Guide 11
Bennett textbook: Chapter 7 except pp. 202-212; Chapter 8; "Summary of Key Concepts" for Chapter 13.
Reading for next lecture:
Bennett textbook: Secs. 8.5, 9.1, 9.6
Study Guide 12
Web Links:
Last modified
January 2024 by rwo
Drawings of stages in the nebular theory from ASTR 161,
University of Tennessee. Computer simulation of protoplanetary disk
by G. Bryden. Velocity curve of 51 Peg from G. Marcy & P. Butler.
Text copyright © 1998-2024 Robert W. O'Connell. All rights
reserved. These notes are intended for the private, noncommercial use
of students enrolled in Astronomy 1210 at the University of
Virginia.